Contact persons: Jan Hynek, Kaplan Kirakci, Kamil Lang
We investigate physicochemical, photophysical, photochemical, and photobiological properties of porphyrinoids and porphyrin-based (nano)materials, e.g., covalent organic frameworks, metal-organic frameworks, nanoparticles, and polymeric nanofibres. In many cases, porphyrin units, which produce singlet oxygen upon visible light irradiation, serve as building blocks of these materials. The variability enables the control of the surroundings of the porphyrin units and prevent their close contacts (aggregation), i.e., provides structural control that, in turn, enables the tailoring for light-induced applications. These molecules and (nano)materials are designed for applications in areas such as photodynamic antimicrobial coatings, photodynamic therapy (especially in the form of nanoparticles), and site-specific oxygen sensing.
1. Singlet oxygen
Singlet oxygen O2(1Δg) is a potent mediator of phototoxicity and is typically generated by energy transfer from the excited triplet states of a photosensitizer to molecular oxygen (Fig. 1). This feature, coupled with the limited diffusion length of the O2(1Δg) in cells (~ 150-190 nm) due to its short lifetime, leads to a high selectivity in the destruction of targeted problematic cells. In addition, O2(1Δg) has strong bactericidal and virucidal properties, which are the bases for a promising method for fighting microorganisms (often resistant) - antimicrobial photodynamic inactivation. Singlet oxygen interacts with cell structures and interferes with different metabolic pathways, preventing the development of resistance of microorganisms towards photodynamic treatment.
References:
- K. Lang, J. Mosinger, D. M. Wagnerová: Photophysical properties of porphyrinoid sensitizers noncovalently bound to host molecules; models for photodynamic therapy. Coord. Chem. Rev. 2004, 248, 321-350.
- K. Lang, J. Mosinger, P. Kubát: Nanofibers and nanocomposite films for singlet oxygen-based applications. Chapter 15, pp. 305 – 321. Singlet Oxygen: Applications in Biosciences and Nanosciences (Vol. 1), S. Nonell, C. Flors (Eds.), European Society for Photobiology 2016. The Royal Society of Chemistry, Cambridge, UK.
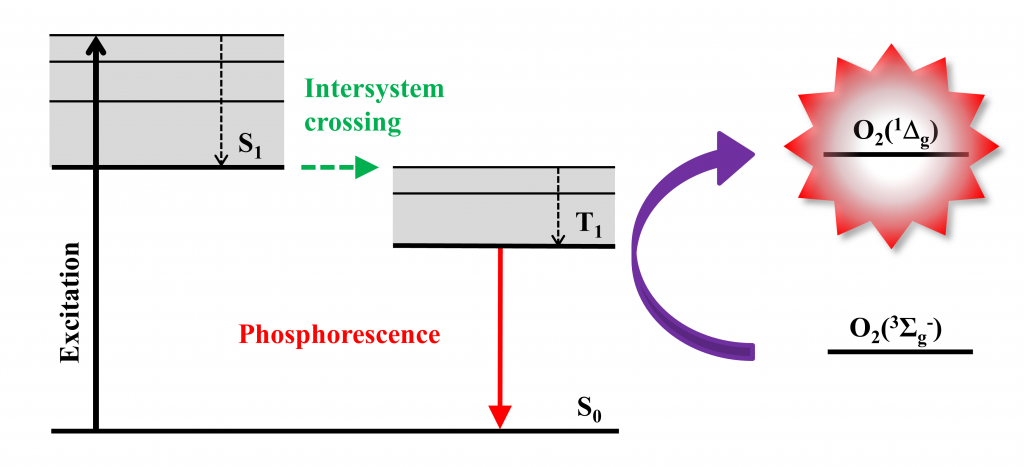
Figure 1. Production of O2(1Δg) by energy transfer from the excited triplet states of a photosensitizer to molecular oxygen. Typical photosensitizers: octahedral molybdenum or tungsten clusters, porphyrins, phthalocyanines.
2. Molecules and (nano)materials
Synthesis of substituted porphyrin derivatives that can serve as apical ligands of {Mo6I8} clusters or that can be incorporated into the structure of Zr(IV)-based MOFs. Substitution of tetracarboxylic porphyrin in a β-position can serve as powerful tool to bring an additional functionality into the structure of porphyrin Zr(IV)-based MOFs (PCN-222, PCN-224). Derivatization of the linkers can improve the affinity of the pore walls to certain guest molecules. The monophosphinate porphyrin represents a ligand with a potential to form a stable-enough coordination bond with a {Mo6I8}cluster complex.
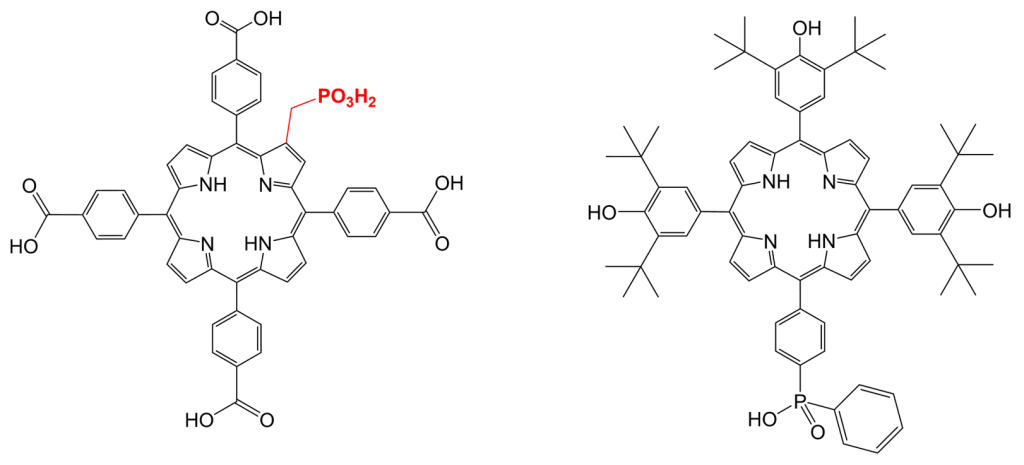
Figure 2. Left: Molecular structure TPPC-PO2H2, a linker for Zr(IV)-based MOFs with additional functionality in a β-position. Right: Molecular structure of a monophosphinate porphyrin derivative suitable for apical coordination to the {Mo6I8} core.
Phosphinatophenylporphyrins. We synthetized and characterized porphyrin derivatives containing four phosphinic functional groups with different substituents on phosphorus atoms (Fig. 3, in blue). The photophysical properties of these porphyrins are not affected by phosphinic groups themselves, and yet the variability of R substituents enables tuning key properties such as hydrophobicity, aggregation, or binding to serum albumin. These porphyrins are attractive for photodynamic applications (Fig. 3).
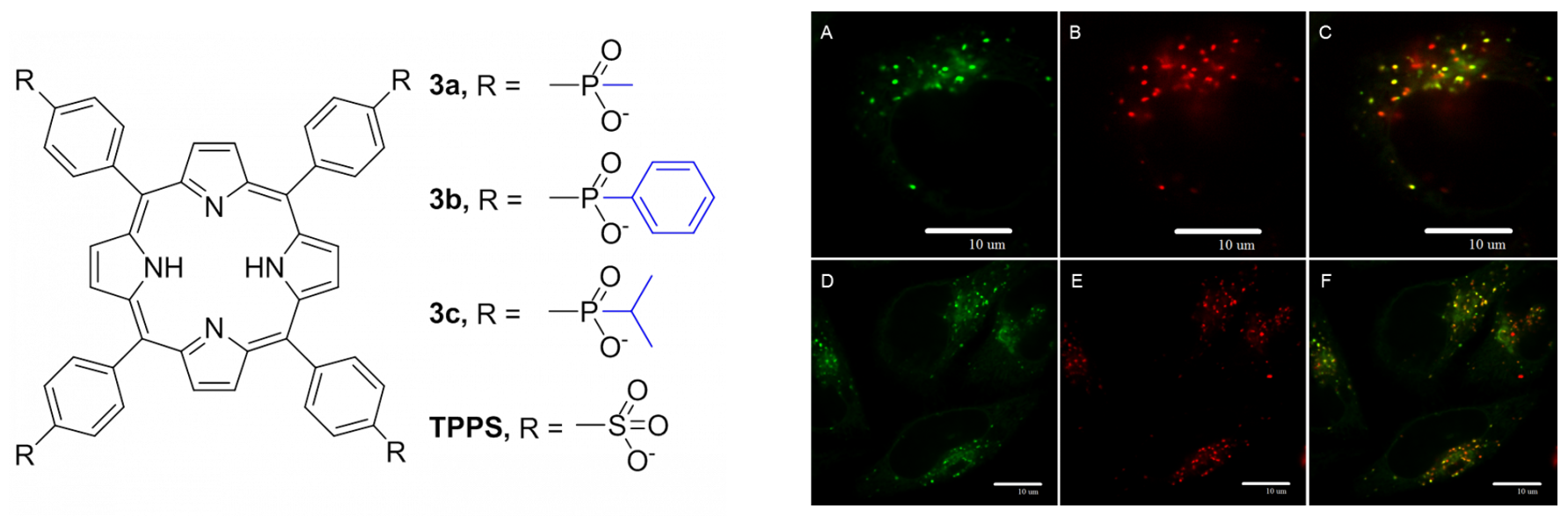
Figure 3. Left: Molecular structures of phosphinatophenylporphyrins. Rigth: Confocal microscopy of HeLa cells incubated with LysoTracker Green (A, D), porphyrin (B, E), and the overlay (C,F, yellow-orange color). It documents that porphyrin is co-localized with lysosomes.
Metal-organic frameworks (MOF). Nanoparticles of hexagonal PCN-222 are powerful in inducing singlet oxygen-mediated apoptosis of cancer cells upon visible light irradiation (Fig. 4). The phototoxicity of the nanoparticles is deactivated after several hours, which is an attractive feature to avoid post-treatment photosensitization issues.
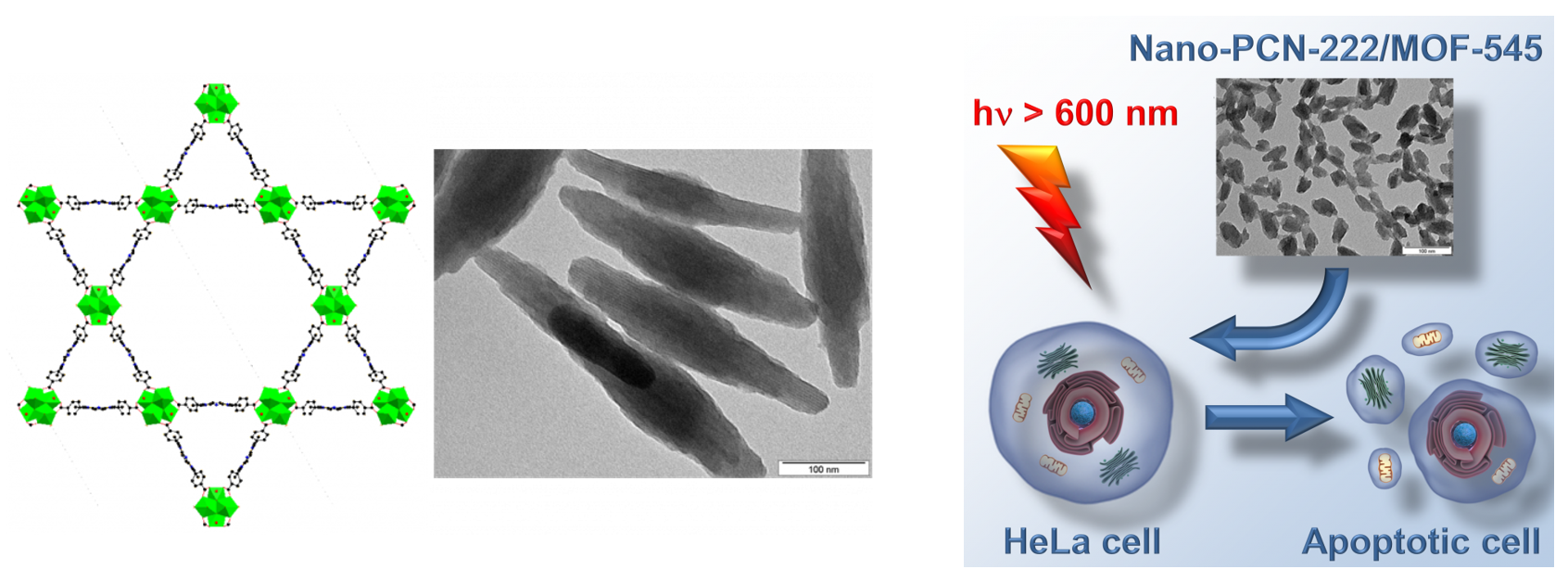
Figure 4. Left: Structure of PCN-222 composed of Zr(IV) structural building units (green) which are connected via porphyrin units. Nanoparticles of PCN-222 under transmission electron microscope. Right: Illustration of photodynamic activity of PCN-222 nanoparticles.
Selected references:
- M. A. Alfred, K. Lang, K. Kirakci, P. Stuzhin, P. Zimcik, J. Labuta, V. Novakova: Subphthalocyanines as fluorescence sensors for metal cations.
Dalton Trans., 2024, 53, 2635-2644. - J. Hynek, M. Koncošová, J. Zelenka, I. Křížová, T. Ruml, P. Kubát, J. Demel, K. Lang: Phosphinatophenylporphyrins tailored for high photodynamic efficacy. Organic & Biomolecular Chemistry 2018, 16, 7274 – 7281.
- J. Hynek, J. Zelenka, J. Rathouský, P. Kubát, T. Ruml, J. Demel, K. Lang: Designing porphyrinic covalent organic frameworks for the photodynamic inactivation of bacteria. ACS Appl. Mater. Interfaces 2018, 10, 8527−8535.
- D. Bůžek, J. Zelenka, P. Ulbrich, T. Ruml, I. Křížová, J. Lang, P. Kubát, J. Demel, K. Kirakci, K. Lang: Nanoscaled porphyrinic metal-organic frameworks: photosensitizer delivery systems for photodynamic therapy. J. Mater. Chem. B 2017, 5, 1815-1821.