Contact person: Bohumír Grüner
Chemistry of cobalt bis(dicarbollide) ion and other metallacarboranes
Among metallacarboranes, particularly the bis(icosahedral) cobalt bis(dicarbollide)(1-) ion (1-) represents a promising platform for advanced applications, due to sandwich structure, delocalization of electrons, two different sites available for chemical modifications, and extremely high chemical, thermal and radiation stability.
Our recent chemistry over last years has been directed to exploring substitution pathways on the cage carbon atoms of the cobalt bis(dicarbollide)(1-) ion with selection of functional groups, which are relevant for further modifications or their presence on the cage introduces interesting stereochemical features. The reactions pathways have been mainly based on metalation CH groups in the first step followed by reactions with electrophiles [1]. Those methods are well known in organic, metallocene and neutral carboranes synthesis. However, due to increased experimental demands, the potential for modifications of the ion had remained unlocked.
Substitutions with selection of functional groups sitting directly on carbon atoms such as: -OH, -N3, -CON3, -NH2, -CN, -I, boronic esters, along with synthesis of new derivatives with aromatic amine group, or tetrazole motif attached via linker have been successfully addressed [1-6]. The carbon substitution enables access to mono and disubstituted compounds and offers a larger scope of structural types and new properties, when compared to the widely studied substitution on boron atoms [1]. The new compounds represent previously inaccessible structural blocks that have been already used in our chemistry focused on the medicinal use [7].
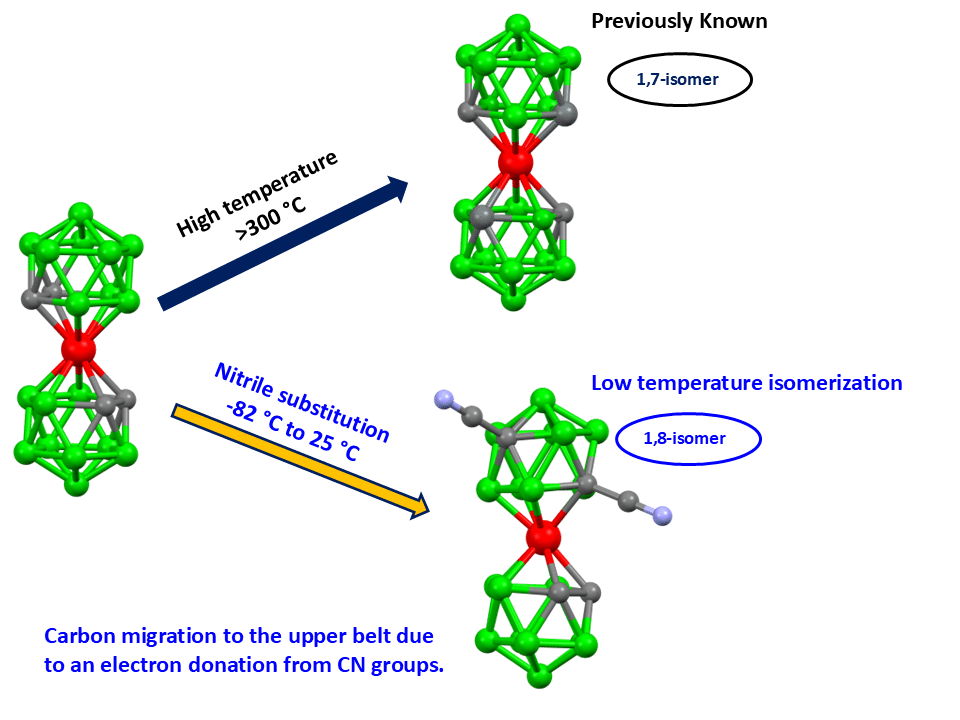
Fig. 1: The figure schematically shows an unique low-temperature isomerization of the cage induced by substitution of two vicinal carbon atoms with nitrile group, which show here a rare electron-donating action.
Studies on reactivity of the substitution groups and their physicochemical properties were carried out in parallel. As we have shown recently, carbon substitution can grossly alter physicochemical properties of directly bound groups, i.e. the acidity constants of NH2 groups significantly decrease being comparable to weaker inorganic acids such as HF [5].
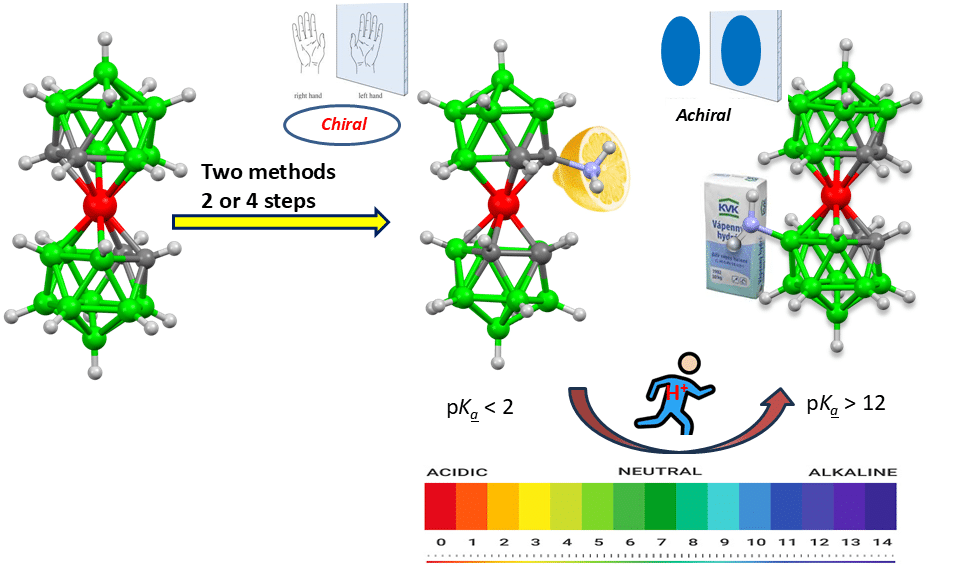
Fig. 2: Effect of structural changes on acid-base properties of cobalt bis(dicarbollide) ion.
The synthetic work has been complemented with studies of structural and stereochemical properties of new compounds. Here we focus on stereochemistry of disubstitution, new types of cage isomerisation, locking the conformation, etc.
Homo and heterosubstitutions with two groups on B and C sites of the boron cage have been studied for the first time and in several directions. In a pilot paper we have shown that it is possible to introduce conformationally restricted geometry due to presence of an oxygen bridge between boron atoms and simultaneously the cage can be substituted carbon atoms with variety of organic fragments via an introduction of alkylazido groups followed with dipolar cycloaddition reactions with a series of model alkynes. Due to bio-orthogonal character of the reaction and enhanced redox potential of the products this method presents promising potential for electrolabelling of biomolecules [3].
![Fig. 3: The figure schematically shows a conformational lock introduced to the cluster by presence of the oxygen bridge between two boron atoms, followed with modification at carbon site(s) with an organic group. This results in a chiral structure. Presence of the polar bridge favourably enhances the electrochemical potential. The organic groups can be introduced directly from cycloaddition reaction or via terminal groups sitting on the triazole ring [3].](https://www.iic.cas.cz/wp-content/uploads/2025/01/NewConc-Fig2.png)
Fig. 3: The figure schematically shows a conformational lock introduced to the cluster by presence of the oxygen bridge between two boron atoms, followed with modification at carbon site(s) with an organic group. This results in a chiral structure. Presence of the polar bridge favourably enhances the electrochemical potential. The organic groups can be introduced directly from cycloaddition reaction or via terminal groups sitting on the triazole ring [3].
Another type of conformationally restrained geometry is represented by unconventional propeller-shaped isomeric molecules, in which one or two ethylene units interconnect boron and carbon positions [4].
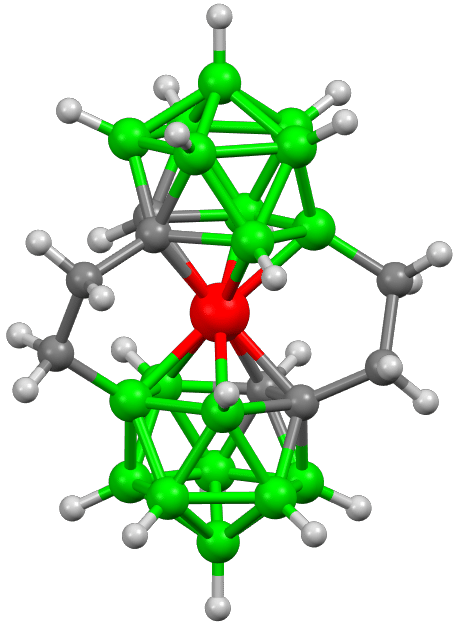
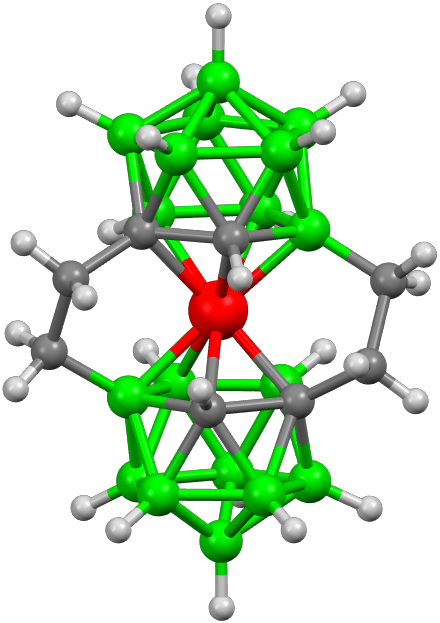
Fig. 4: Molecular structure of isomeric ions containing two ethylene bridges between carbon and boron atoms. The presence of bridges leads to restricted rotation of the two dicarbollide ligands around the central Co(III) atom and asymmetry of the whole scaffold [4].
To the most striking stereochemical features belongs the planar or axial chirality induced by asymmetric substitution(s) on the cobalt bis(dicarbollide) cage. The progress in this field has been enabled by development of advanced analytical and semi-preparative HPLC separations of boron cluster anions on Chiral Stationary Phases (CSP) in collaboration with group of Assoc. Prof. R. Kučera from Faculty of Pharmacy, Charles Univ., HK. Results on successful enantioseparations of cobalt bis(dicarbollide) (1-) ion derivatives has been described in several recent articles [3, 5, 8-10].
The new compounds are of interest as building blocks for various purposes such as material chemistry and the medicinal use. The chiral molecules can be further applicable as 3D chiral selectors or chiral auxiliaries in asymmetric synthesis. Indeed, some molecules resemble BINOL or BINAP, however providing different steric and bonding interactions.
References:
- L. Pazderová, E. Z. Tüzün, D. Bavol, M. Litecká, L. Fojt and B. Gruner, Molecules, 2023, 28, 67.
- S. El Anwar, Z. Ružičková, D. Bavol, L. Fojt and B. Grűner, Inorg. Chem., 2020, 59, 17430-17442.
- S. El Anwar, L. Pazderova, D. Bavol, M. Bakardjiev, Z. Ruzickova, O. Horacek, L. Fojt, R. Kucera and B. Gruner, Chem. Commun., 2022, 58, 2572-2575.
- J. Nekvinda, D. Bavol, M. Litecká, E. Z. Tüzün, M. Dusek and B. Grüner, Dalton Trans., 2024, 53, 5816-5826.
- Z. E. Tüzün, L. Pazderová, D. Bavol, M. Litecká, D. Hnyk, Z. Růžičková, O. Horáček, R. Kučera and B. Grűner, Inorg. Chem. , 2024, 63, 20600-20616.
- E. Z. Tüzün, D. Hnyk, D. Bavol, M. Litecká, J. Fanfrlik and B. Gruner, Dalton Trans., 2024, 53, 18462-18466.
- L. Pazderová, E. Z. Tüzün, D. Bavol, M. Litecká, L. Fojt and B. Grűner, Molecules, 2023, 28, 67.
- O. Horáček, M. Papajová-Janetková, B. Grüner, L. Lochman, P. Štěrbová-Kovaříková, R. Vespalec and R. Kučera, Talanta, 2021, 222, 9.
- O. Horacek, J. Marvalova, K. Stilcova, J. Holub, B. Gruner and R. Kucera, J. Chromatogr. A, 2022, 1672, 11.
- O. Horacek, L. Novakova, E. Tuzun, B. Gruner, F. Svec and R. Kucera, Analytical Chemistry, 2022, 94, 17551-17558.